Time-of-Flight Secondary Ion Mass Spectrometry
TOF-SIMS (Time-of-Flight Secondary Ion Mass Spectrometry) is a high-resolution, if required, imaging analysis method for characterizing solid surfaces. This technique is a variant of TOF mass spectrometry. It provides information on the local chemical composition of the sample with a lateral resolution in the sub-micrometer range by analyzing secondary ions. The scanning of the primary ion beam over the sample surface enables the mass distribution and composition of the sample surface to be visualized in a so-called mass image. In contrast to nanoscale SIMS, ToF-Sims does not work with a sector field mass spectrometer, but with time-of-flight measurements.
While quadrupoles were used in the first SIMS devices, they are now considered obsolete due to the sequential interrogation method of quadrupole MS. Magnetic sector field mass spectrometers also have system-related limitations, meaning that time-of-flight-based methods are becoming increasingly popular, as complete mass spectrum can be recorded with each pulse of the system. TOF measurements are based on the separation of accelerated ions in an electric field according to their mass-to-charge ratio based on the time of flight along a field-free drift path.
ToF-SIMS is particularly suitable for analyzing the uppermost atomic layers and also enables hyperspectral, three-dimensional imaging for spatially resolved material analyses of surfaces, thin layers, and interfaces of the samples under investigation (depth profiling). Both conductive and insulating materials can be analyzed, the only requirement being that the sample be placed in a vacuum.
ToF-SIMS offers excellent specificity for a wide range of microstructure analyses of solids, liquids, and pasty materials. Although this is a destructive method in which the analyzed surface is partially degraded, it is considered to be virtually non-destructive due to the low number of primary ions per unit area.
Mode of operation:
In ToF-SIMS analysis, primary particles (usually either cations or anions of inert gas) are fired at the sample surface (with kinetic energy in the higher KeV range up to the MeV range), whereby the surface is usually processed in a grid. The ion beam itself can be focused on an area with a diameter of fewer than 0.1 micrometers. In some cases, the ions pass through a Wien filter and therefore have the same speed. On impact (if the energy introduced is high enough), the ions penetrate the sample and transfer their kinetic energy to the particles in the first layer, whereby this momentum transfer disrupts the lattice atoms (or molecules) of the sample. This triggers a collision cascade in the sample material, whereby some particles receive sufficient energy to be emitted from the sample material (sputtering). The ion yield varies by several orders of magnitude for the different elements. The yield of secondary ions produced during the sputtering of organic and inorganic materials is very low in most cases, with the most frequently emitted fragments being neutral particles.

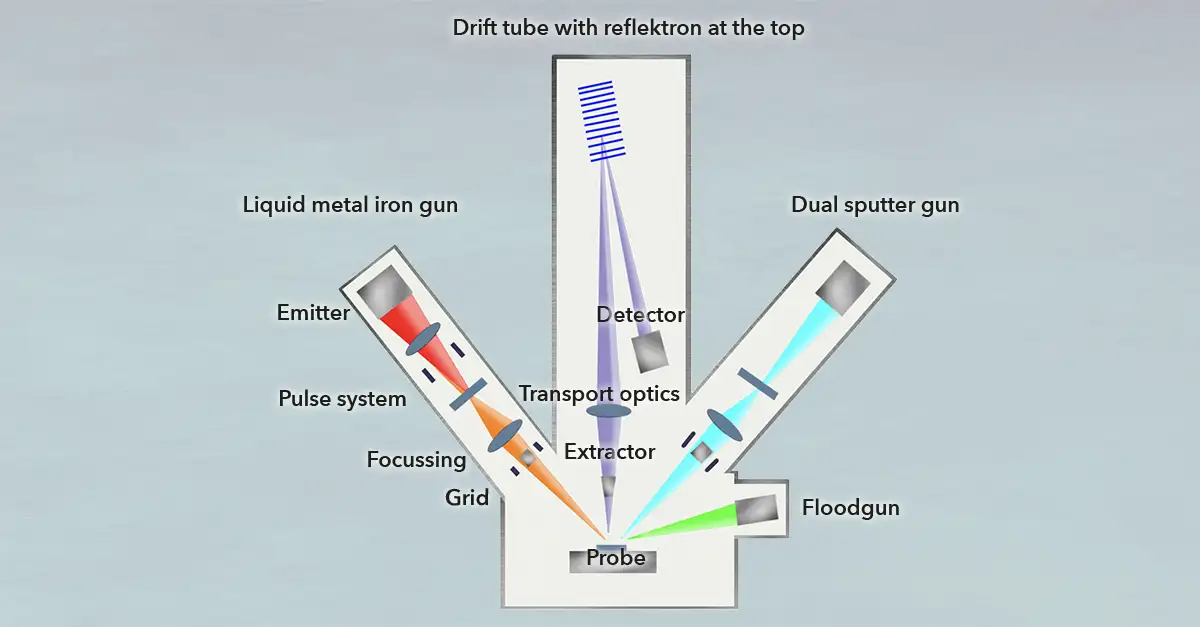
Description of the elements of a ToF-SIMS device:
- Primary ions are often generated using electrospray ionization. A liquid metal iron source (liquid metal iron gun, LMIS, LMIG) is used for this, which usually has a probe diameter in the micrometer range. An analyzer solution is passed through a metal capillary, to the tip of which a voltage is applied. This results in the formation of an electric field between the capillary and a counter electrode, which generates a focussed ion beam. Ga ions are suitable for analyzing low-mass areas (mainly elements), while Au and Bi ions are suitable for high-mass areas (mainly molecules).
- Secondary ions emitted from a solid surface have an energy distribution of several eV to several dozen eV and a large emission angle, which can lead to differences in the arrival time at the detector even with the same ion mass. This problem can be countered with a Wien filter behind the ion source, which enables mass separation through momentum deflection. This consists of a static deflection (static high voltage) for the ion beam and a pulse generator (pulsed high voltage) arranged at an angle of 90° and helps to ensure that only those particles with a certain velocity pass through the filter.
- In the so-called buncher, an additional voltage is introduced with a time delay so that the primary ion pulses are further focussed. This measure leads to an efficient reduction in the pulse width.
- The primary ion beams hit the sample in the target and trigger a shock cascade (sputtering) in the uppermost layer of the sample material.
- For charge equalization when measuring insulating materials, the primary ions are simultaneously irradiated by a flood gun with pulsed low-energy electrons to prevent the sample from being charged by the primary ion irradiation.
- For the evaluation of deeper areas, a sputter ion gun can be used in addition to the primary ion gun to specifically remove material layers. In general, oxygen (02) and cesium (Cs) ions are used for the depth profile analysis of inorganic materials such as metals and semiconductors. Fullerenes (C60) and argon gas cluster ions (Ar-GCIB), on the other hand, are used for organic materials.
- The secondary ions are accelerated into the drift tube of the time-of-flight mass spectrometer. The reflectron at the upper end of the drift tube increases the distance of the flight path.
Laser SNMS:
The sensitivity of ToF-SIMS measurements can be further increased using laser SNMS. In this process, the sputtered neutral species are post-ionized with a laser pulse, which significantly increases the signal-to-noise ratio of the ToF-SIMS measurement. However, the detection of large organic ions may be more difficult due to photo fragmentation. Laser-SNMS offers higher sensitivity for neutral atoms and is particularly suitable for precise quantitative analyses, while TOF-SIMS is particularly advantageous in qualitative chemical surface analysis and imaging.
Data acquisition:
Detectors used for ToF-SIMS are:
- MCPs
- Sector field detectors
- Orbitraps
- Hybrid photodetectors
- Secondary electron multipliers (SEV)
- Semiconductor detectors such as SPADs, APDs
Typically, the intensity distribution of a selected mass is displayed in a mass image, whereby the materials present are deduced from the interpretation of the resulting mass spectra. The count rate for each pixel of the image is indicated by a brightness value. Modern ToF-SIMS devices achieve maximum lateral resolutions of up to 60 nm for geometrically flat, conductive samples. This limit is given by the diameter of the primary ion beam.

Applications:
ToF-SIMS can be used to examine a wide variety of materials, such as metals, ceramics, glasses or plastics, conductive and insulating substrates, powders, or fibers. Smooth surfaces are an advantage.
- In materials science, TOF-SIMS is a frequently used technique for characterizing thin films and nanomaterials, material surfaces, and interfaces. For example, metals, alloys, ceramics, optical components, coatings, paper, paints, diffusion processes, catalytic solids, or corrosion products are analyzed.
- In quality assurance for industrial processes, ToF-SIMS measurements can be used to detect contamination, check layer homogeneity in composite materials and, if necessary, detect layer closure. One of the most common applications is the clear identification of organic compounds on surfaces (e.g. silicones/silicone oils, PDMS, greases/oils, or adhesive residues). A wide variety of polymer layers can also be analyzed and, for example, aging processes in polymers can be detected. The characterization of catalytic materials, the investigation of adsorption and desorption processes, and the analysis of chemically modified surfaces (e.g. the analysis of surface coatings on fabrics) are just as possible as the identification of inorganic or metallic impurities and the determination of the surface composition of alloys.
- In the semiconductor industry, impurities, defects, and layer compositions are examined to ensure the control and optimization of production. For example, thin oxide and nitride layers are identified at the atomic level as part of the impurity analysis, and depth profiles of dopants can be created. Surface mapping using ToF-SIMS is used, for example, to check bond contacts and coatings.
- To investigate the distribution of active ingredients and excipients in the pharmaceutical industry, this technique is used to determine the distribution of drugs in tissues (e.g. as part of the investigation of drug release systems). Coatings on tablets can also be characterized as part of quality control.
- TOF-SIMS is primarily used by bioscientists to investigate biomolecules in tissues and cells so that lipid and protein distributions can be analyzed, for example.
- In geosciences, ToF-SIMS is primarily used to analyze geological processes, minerals, and rocks, e.g. when examining extraterrestrial samples.
- Forensics uses this examination method to analyze traces of explosives, drugs, and other chemical residues and identifies such traces at crime scenes.
- Tof-SIMS is also used to examine color pigments and materials in works of art, e.g. to determine the aging and preservation of historical artifacts.
- Environmental scientists use ToF-SIMS to analyze pollutants and their distribution in the environment, e.g. in soils and sediments.
Advantages of ToF-SIMS:
- If SIMS analyses are carried out using time-of-flight measurements, the available mass range is extended by a factor of about ten compared to quadrupole-based measurements. This makes it possible to recognize very small quantities of materials on the surface of the sample.
- High sensitivity: Thanks to its high spectral mass resolution ToF-SIMS can detect very low concentrations of elements and molecules, often in the range of a few ppm or even in the sub-ppm range. This is the case for almost all elements, even very light elements such as hydrogen, lithium, and boron. Due to the very high surface sensitivity depth profiles with a depth accuracy of < 1 nm and a measurement speed of up to 10 µm/h are achievable. As a result, in addition to elements, chemical compounds can also be detected.
- molecular information via fragment ions/fragment ion patterns
- There is no theoretical upper mass limit for SIMS analyses.
- All masses can be detected within one analysis cycle.
- High lateral resolution: This technique enables the investigation of very small areas (down to the nanometre range < 100 nm) and can create very accurate 2D or 3D images of the sample surface and its chemical composition.
- Wide range of applications: The measurement can be applied to a variety of materials, including organic and inorganic samples. Of all current surface analysis methods, SIMS is the only method that is sensitive to all elements.
- No sample preparation is required: In many cases, the analysis can be performed without extensive sample preparation, preserving sample integrity.
- Contamination-free analysis of cross-sections using dynamic ToF-SIMS: Due to the very low primary ion currents used, which generally only remove the top 1-2 atomic layers, surface analysis is almost non-destructive.
Limitations of ToF-SIMS:
- A disadvantage of ToF-SIMS is that the fragmentation rate of the target molecules can be quite high in individual cases. This is because analyzing organic deposition species, for example, results in extremely complex mass spectra with an unavoidable background level across the entire mass spectrum. This may make it difficult to identify the original molecules.
- The formation of secondary ions depends to a large extent on their chemical environment. Due to the dependence of the ionization probability on the surrounding matrix, ToF-SIMS is only a semi-quantitative method. Quantification is only possible by comparing the spectra with standard samples of known composition.
- The sensitivity fluctuations in SIMS are high. The electropositive elements usually have a higher sensitivity in SIMS with positive primary ions and the electronegative elements have a higher sensitivity in SIMS with negative primary ions.
- A reduction of organic surface species may occur due to the release of secondary electrons. At very low ion doses, however, this effect is usually negligible.