TOF mass spectrometry
In many ToF-MS units, cronologic TDCs or ADCs are used to measure precisely the arrival of single ions. From the arrival time, the ion’s time-of-flight is deduced, from which the mass-to-charge ratio of the detected particle can be determined. A crucial factor for a successful measurement is the extremely low cycle-to-cycle jitter of our TDCs and their very low multiple hit detection dead time.
When it comes to finding out the composition of unknown substances, mass spectrometry is used in most cases. This applies to the checking of chemical compounds during their production and to the analysis. For this purpose, the mass of individual atoms or molecules of a sample is precisely determined. ToF mass spectrometry (ToF-MS) offers advantages in terms of accuracy and sample throughput in automated measurement methods, e.g. for high-throughput screening tools. Modern mass spectrometers often work in several stages (tandem MS, MS / MS). Particularly precise are measurements in which isolated ions are examined with a subsequent ToF measurement.
The field of ToF mass spectrometry comprises a large number of sophisticated measurement schemes that have been optimized for their respective area of application. In many analyzers, for example, the actual ToF measurement is preceded by a coupled chromatographic separation of the sample. The ToF-MS is coupled with gas chromatography (GC-MS), liquid chromatography (LC-MS), ion mobility spectrometry (IMS-MS), or with capillary electrophoresis (CE-MS) or a combination of such separation approaches. This initial separation is particularly helpful when evaluating very complex samples, e.g. in food analysis. In all of these cases, however, at the heart of the devices and the key to their precision is ultimately the time-of-flight analyzer.
Function & measurement setup:
In a time-of-flight mass spectrometer, ionized atoms or molecules of a sample are separated on the basis of their mass-to-charge ratio during the passage of a flight tube, so that ultimately their mass can be determined from a measurement of their time of flight. When entering the analyzer (which consists conceptually of an electric field with known strength), the ions obtain kinetic energy proportional to their charge. After the passage of the electric field region, all ions have the same kinetic energy. However, their flight times depend on their mass such that light ions are detected earlier than heavy ions. This is the case with ions of the same charge. The measured time of flight (ToF) is recorded and is proportional to the square root of the mass of the molecule.
On the most basic level, time-of-flight analyzers essentially consist of a tube that contains a high vacuum and is equipped with an extremely fast ion detector at its end.
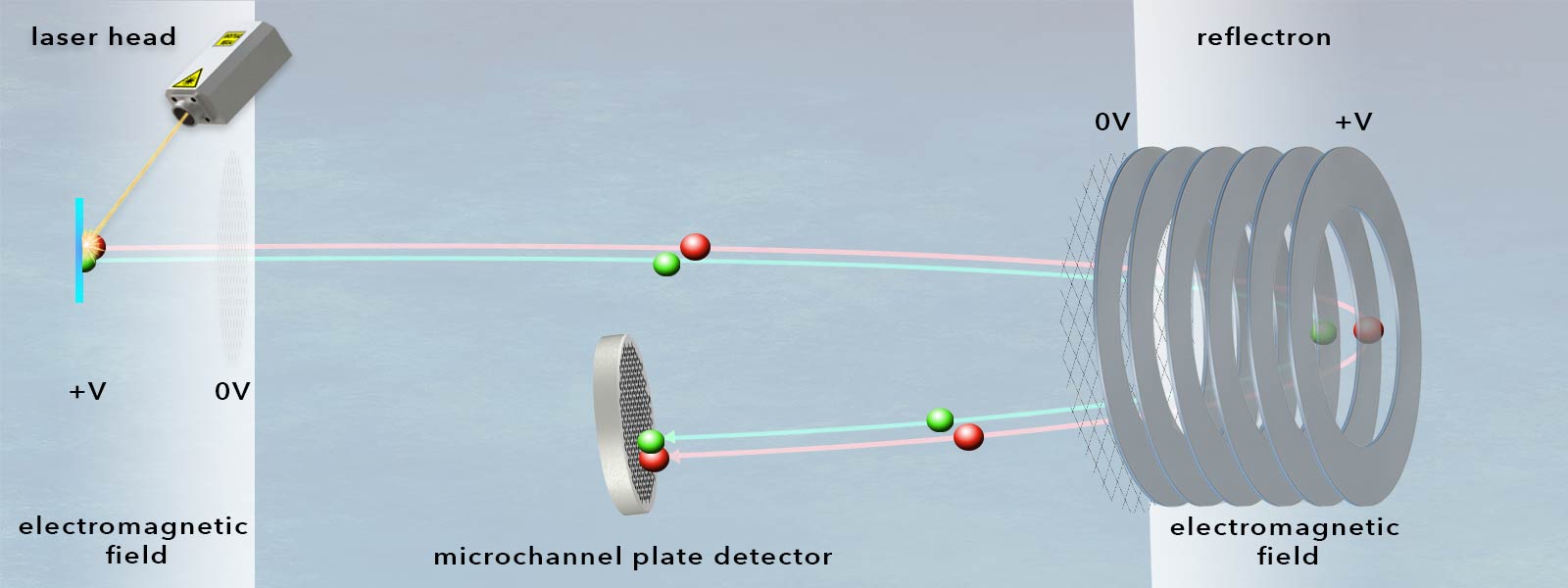
How is ionization of the sample implemented?
Depending on the area of application and the chemical, biological or physical properties of the examined sample, different ways of its ionization may be considered. If the sample is not already in the gas phase, it must be first be vaporized or nebulized. The sample substance is then typically ionized by particular collision (electron impact ionization), protonation (chemical ionization), or by means of strong laser light. Ideally, this process yields singly charged ions.
Here is a brief overview of various ion sources:
- Electron ionization (EI, formerly known as „electron impact ionization“ and electron bombardment ionization) is the classic way of ionization in which energetic electrons interact with solid or gas-phase atoms or molecules. An electron gun shoots electrons specifically at the sample, from which ions are generated directly. By proper tuning of the electron gun properties, exactly one electron is released from the sample molecules or atoms. Thus, the ions produced in this way may have different masses, but are for the most part, of the same charge. EI imparts a large amount of energy to the sample resulting in extensive fragmentation. This hard ionization method is useful for the analysis of volatile, thermally stable, low-mass organic compounds.
- The MALDI-TOF (matrix-assisted laser-desorption-ionization) method is very common here. This uses laser light in conjunction with a chemical matrix to ionize the sample. The advantage of MALDI-TOF MS lies in the high throughput with which a wide variety of samples can be measured. The disadvantage here, however, is that the ionization efficiency varies depending on the type of analyte and is sometimes might decrease rapidly. Plus the laser source can possibly be harmful to light-sensitive samples.
- The milder ionization conditions of electro-spray ionization (ESI) offer the possibility of examining unstable and light-sensitive samples more gently. To do this, the sample is dissolved in a very volatile liquid (water, ethanol, methanol). This analyte solution is then pressed through very fine metal capillaries at very high pressure so that an aerosol is formed. The tip of the needle is biased with a positive high voltage. When leaving the needle, the droplets of the aerosol absorb hydrogen ions from the protic solution. The remaining solution evaporates and the ionized droplets are attracted to the negatively biased part of the measuring device and thus accelerated.
Please note: The mass-to-charge ratio of the sample is changed by this type of ionization. When examining the time of flight, the mass of the additionally absorbed protons must be taken into account. More precisely, there are several positive charge states for each constituent of the analyte solution, so that it appears several times in the mass spectrum. The analysis of the measured data is, therefore, more complex.
- Photoionization is based on ionization through the photoelectric effect. The first laser-induced photoionization in the gas phase was observed as early as 1970. This process was later called multi-photon ionization (MPI). Organic molecules allow typically for a resonant MPI, so-called resonance-enhanced multi-photon ionization (REMPI). Today, there is extensive research on the use of photoionization in mass spectroscopy, e.g. for the investigation of nanoparticles produced by combustion. In Atmospheric Pressure Photoionization (APPI), for example, the initial ionization occurs through photoionization, whereby a light-absorbing dopant is added to the sample flow. Since comparatively few ions are generated during photoionization, this technique is considered so soft that it is ideal for studying organic molecules. This sampling technique is ideally suited for combination with a time-of-flight mass spectrometer.
- Via field ionization, strong electric fields in the immediate vicinity of sharp pins/tips, thin wires, or sharp edges may also cause ionization. The ionization takes place on the surface of the field emitter on which an electronically highly excited atom or molecule spontaneously loses an electron without any further interaction. It is therefore a very gentle ionization method (known as “field desorption”) gaining importance nowadays again, especially in combination with very accurate TOF measurements.
- Fast atom bombardment (FBA) involves shooting an accelerated beam of atoms or ions at a small metal target from an atom or ion gun. The metal target is mounted on a probe and loaded with a viscous liquid matrix (usually 3-Mercaptopropane-1,2-diol or m-NBA) in which the sample to be analyzed is dissolved. When the atom or ion beam collides with the matrix, many surface molecules of the sample are ionized and sputtered into the high vacuum. Compared to electron impact ionization, FAB is a “gentle” ionization process that is suitable for a large number of compounds, provided the sample is soluble in the matrix.
- In inductively conducted plasma mass spectroscopy (ICP-MS), a high-frequency electromagnetic field is used to generate plasma in an argon gas flow, in which a sample aerosol is heated to temperatures up to 10,000 ° C. In this process, the sample components are vaporized, broken down into their atomic components, and ionized. Coupled with TOF measurements, this ionization method offers a very good dynamic range and is considered a robust method in inorganic element analysis for element trace analysis of heavy metals such as mercury, lead, or cadmium.
Since extensive further developments are taking place with regard to ionization techniques, this list does not claim to be exhaustive.
Which detectors are used?
The separated ionic components are characterized by measuring their time of arrival at the end of the flight path using a detector. Several types of detectors are available for mass spectrometers.
- The Faraday cup is the simplest form of a detector. It collects ions and provides information on the detected current. One advantage of the Faraday cup is its reliability and the ability to measure the current directly as an absolute value. Because of its low detection sensitivity, this system is still used exclusively in isotope mass spectrometry.
- Classic photomultiplier tubes are electron tubes that pick up weak light signals (up to individual photons) and amplify them.
- Avalanche photodiodes are highly sensitive, fast photodiodes and form the basis for modern silicon photomultipliers (SiPM). They use the internal photoelectric effect to generate charge carriers and the avalanche effect for internal amplification.
- Hybrid photodetectors detect very small amounts of light by combining the properties of photomultipliers and avalanche photodiodes.
- Daly detectors are high-performance ion counters with a very high dynamic range that are used to analyze gases. The construction of a Daly detector enables the photomultiplier used to be separated from the high vacuum of the mass spectrometer, which prevents possible contamination and increases the life span of the PMT.
- Channeltron detectors interact with electrons, positive and negative ions, hard and soft X-rays generating an electron avalanche from a primary particle.
- Microchannel plate detectors (MCP) detect electrons, ions, or high-energy photons. They work with particularly low noise and also provide spatial information if required.

How are the data recorded in TOF mass spectrometry evaluated?
If an atom in a compound exists as naturally occurring isotopes, these will also be visible in the mass spectrum when using a high-quality ToF mass spectrometer, because the isotopes have different mass/charge ratios. This complexity offers additional possibilities for the determination of sample contents when evaluating the mass spectra. In order to identify sample contents, the following data are usually determined in a ToF mass spectrometer:
- The accurate mass of the ionized compound. The accurate mass of the ionized compound. In addition to the overall design of the mass spectrometer and the requirements that need to be met by the vacuum, accurate calibration is of the device is vital for precise measurements. In many cases, this calibration is performed together with the actual sample measurement. For example, a calibration solution with known content is used for calibration.
- The relative abundance of isotopes The relative abundance of isotopes can serve as a characteristic fingerprint for a given substance and also allows further information to be gathered about the content of the sample.
- The so-called isotope spacing. The isotope spacing which reflects the isotope distribution is also a characteristic feature of a substance.
The advantages of ToF mass spectrometry compared to other mass spectrometric methods:
- ToF-MS enables the simultaneous measurement of the mass-to-charge ratios of all ions almost without restriction on the mass range.
- Thanks to their enormous mass resolution, ToF measurements offer particularly precise detection options.
- In ToF measurements, no important information about sample contents is usually lost, so that even particularly complex mixtures can be characterized.
- Since even isotopes appear separately in the mass spectrum, ToF measurements facilitate the identification of analytes and thus the interpretation of the measurements.
Author: Uwe Thomaschky